Research Article
Şeyma Korkmaz
Şeyma Korkmaz
Department of Chemistry, Necmettin Erbakan University, 42090, Konya, Turkey
E-mail: seymakrkmz5@gmail.com
İbrahim Ender Mülazımoğlu
İbrahim Ender Mülazımoğlu
Corresponding Author
Department of Chemistry, Necmettin Erbakan University, 42090,
Konya, Turkey
E-mail: iemulazimoglu@erbakan.edu.tr,
Tel: +90-3323238220, Fax: +90-3323238225
Ayşen Demir Mülazımoğlu
Ayşen Demir Mülazımoğlu
Department of Chemistry, Necmettin Erbakan University, 42090, Konya, Turkey
E-mail: admulazimoglu@erbakan.edu.tr
Abstract
In this research, PGE/CTP/MWCNT electrode was prepared by modifying a mixture of coal tar pitch (CTP) and multi-walled carbon nanotube (MWCNT) onto the pencil graphite electrode (PGE) surface. Differential pulse voltammetry (DPV), square wave voltammetry (SWV) and square wave adsorptive stripping voltammetry (SWAdSV) techniques were used independently for standard dopamine (DA) determination. For the determination of DA on the sensor electrode surface, pH study was performed using BR buffer solution and phosphate buffer solution (PBS). For the determination of DA at different concentrations, DPV, SWV and SWAdSV techniques were used in PBS at pH 7.0. Studies were also carried out to determine time and concentration parameters using SWAdSV. Calibration graphs were drawn between 1 mM and 1 µM on the PGE/CTP/MWCNT electrode surface for the DA solutions. As a result of the studies, it has been determined that DA can be analyzed with high sensitivity, fast, and low cost by using the PGE/CTP/MWCNT sensor electrode.
Abstract Keywords
Dopamine, sensor electrode, PGE/CTP/MWCNT, SWAdSV SWV, DPV.
1.
Introduction
The human brain,
which is known as the most complex object in the known universe, is today characterized
as the last frontier of science in many ways. So much, in a situation where
scientists have not yet fully understood even a single cell, there are a
hundred billion neurons in our brain and close to quadrillion connections
between them. The importance of dopamine (DA, 3,4-dihydroxyphenyl ethylamine)
in central nervous system function and pathology has prompted scientists to
investigate how the neurotransmitter is controlled in the brain extracellular
microenvironment. In the human brain, DA, a neurotransmitter from the
catecholamine class, plays an important role in the renal, cardiovascular,
central nervous and hormonal systems. Abnormalities in DA concentrations have
been associated with various neurological disorders such as Parkinson, epilepsy,
and schizophrenia. DA is also available as an intravenous drug that acts on the
sympathetic nervous system to produce effects such as increasing heart rate and
blood pressure. For this reason, the observation and quantification of DA in
vivo/in vitro is of great importance in clinical medical applications [1-3].
DA is one of the catecholamine and phenethylamine families, an organic compound that has a molecular formula C8H11NO2 and skeletal formula as shown in Fig. 1. DA is produced in the human brain and constitutes about 80% of catecholamines found in the brain. Abnormal levels of DA that are produced in the brain are responsible for some disorders, diseases and negatively affect the body’s balance. Because of its function as a neurotransmitter and messenger among nerve cells. As a chemical messenger, DA affects brain processes that emotional response, control movement and ability to experience pain and pleasure [4]. It’s important to determine DA to protect humans from dangerous illnesses [5].
Figure 1. Molecular structure of dopamine
DA concentration in some human fluids (blood serum and urine) are too small, as well as the complex matrix of the biological samples that contain DA make its determination more complicated and drive analyst to do sample pretreatment to obtain a good quantitative result. Some interferences in real biological samples have the same chemical properties compared to DA that’s open an approach to determine DA concentration according to its electrochemical, biological, or optical properties making determination of DA selectively in a small amount reachable. Determination of DA in biological samples is challenging because of the high concentrations of ascorbic acid that join the low concentrations of DA [6].
According to some previous literature DA determined quantitatively in mouse brain striatum sample in presence of serotonin, 3,4-hydroxyphenylacetic acid, 3-methoxytyramine and homovanillic acid as a sample matrix by HPLC-MS method after derivatization using ethyl chloroformate [7], and by fluorescence-based method in human urine samples after reaction with resorcinol [8], also determined by spectrophotometric method in some pharmaceutical formulations, either by direct measurement of the sample in the UV region at 280 nm or after oxidation with potassium permanganate to obtain a green product that absorbed at 610 nm [4].
Depending on the DA electroactive characteristics and its ability to oxidize [4], DA has been determined by electrochemical methods using fabricated selective electrodes and modified electrochemically or physically. DPV [8, 9], SWV [10,11] and cyclic voltammetry (CV) [12] techniques were used to determine DA in human serum that contains electrode material, modifiers type and other conditions used in the determination.
Electrochemical methods that depend on the modified electrodes have been employed in a variety of analytical applications. Modification of carbon surfaces by electrochemical techniques is an essential objective in material science and electrochemistry. The chemical sensor electrode obtained by electrochemical methods has been frequently used in the quantitative determination of organic and inorganic species in recent years. Carbon electrodes are generally used because of low cost, speed, low equipment, chemical inertness, low background current, wide potential window and minimum sample pretreatment required prior to analysis in electrochemical techniques [13-15]. The purpose of this study was to examine the usability of PGE/CTP/MWCNT sensor electrode in the determination of DA by DPV, SWV and SWAdSV techniques.
2. Materials and methods
2.1 Chemicals
All chemicals were purchased from Fluka, Merck, Sigma-Aldrich and Riedel. All chemicals of analytical purity were used without any further purification. Ultra-pure quality of water with a resistance of 18.2 MΩ cm (MP MINIPURE purification system, DEST UP, USA) was used to prepare all aqueous solutions, measurements, cleaning of the glassware and polishing the electrodes. As in the literature, the Britton–Robinson (BR) buffer solutions of pH 1.81–11.98 at different pH were prepared [16, 17] by mixing H3PO4, CH3COOH and H3BO3 completed to the level by using ultrapure water and PBS (pH 6.0-8.0) by mixing Na2HPO4 and KH2PO4 were used as the supporting electrolytes. The pH adjustments were performed by dropwise addition of 0.2 M/1 M NaOH.
2.2 Electrodes and apparatus
In our experiments, all electrochemical and spectroelectrochemical measurements were performed with a voltammetric analyzer GAMRY Reference 600+ potentiostat/galvanostat/ZRA with C3 cell stand. The one compartment cell with the three-electrode was used for the electrochemical workstation through a C3-stand from BAS (Bioanalytical Systems, Inc., BAS in USA). A PGE/CTP/MWCNT electrode was employed as working electrode. A platinum wire electrode from BAS Model MW-1032 was employed as an auxiliary electrode. All the cell potentials were measured with respect to Ag/AgCl/3 M KCl reference electrode from BAS Model MF-2063. SWV, DPV and SWAdSV were used with PV220 Pulse Voltammetry Software (PA, USA). pH-meter with a combined glass pH electrode (VWR pH enomenal) was used to measure the pH value in the aqueous solutions.
2.3 Preparation of carbonized PGE/CTP/MWCNT electrode
PGE/CTP/MWCNT electrode was prepared by modifying a mixture of coal tar pitch (CTP) and multi-walled carbon nanotube (MWCNT) on the pencil graphite electrode (PGE) surface. PGE/CTP/MWCNT was prepared with a MWCNT/CTP (in acetone) dopped PGE. The amount of MWCNT and CTP was adjusted as 1% (w/w). 10 g MWCNT and CTP composite was carbonized in a N2-atmosphere tube furnace (Protherm, Turkey) with a heating rate of 10 ○C min-1 to reach 1000 ○C. This was followed by the annealing of the materials at 1000 ○C in N2 atmosphere for 1 h. The black material transformed into metallic grey [18,19].
3. Results and discussion
3.1 The use of PGE/CTP/MWCNT electrode as a sensor electrode
DA concentration in some human fluids (blood serum and urine) are too small, as well as the complex matrix of the biological samples that contain DA make its determination more complicated and drive analyst to do sample pretreatment to obtain a good quantitative result.
This study was put forth and proved that obtained PGE/CTP/MWCNT electrode is used for the quantitative determination of DA. For this purpose, firstly the sensitivity of PGE/CTP/MWCNT electrode to DA was investigated using DPV and SWV techniques. DPV measurements were performed in a three-electrode cell, in PBS at pH 7.0. Current measurements were carried out using DPV in the potential range between -0.4 and +0.5 V. The following instrumental parameters were utilized to record the differential pulse voltammograms: Pulse size 50 mV s–1 and frequency 25 Hz. SWV measurements were performed in a three-electrode cell, in PBS at pH 7.0. Current measurements were carried out using SWV in the potential range between -0.4 and +0.5 V. The following instrumental parameters were used to record the square wave voltammograms: Pulse size 50 mV s–1 and frequency 25 Hz. All the electroanalytical measurements were applied at room temperature. Measurements were taken using DPV and SWV techniques to decide which technique to continue with the study. The obtained DPV and SWV voltammograms are given in Fig. 2A and Fig.2B. In studies using DPV and SWV techniques, the peak currents are 27.88 and 71.17 µA, respectively. The studies should be continued with SWV technique was decided (Fig. 2B). In later studies, voltammograms were taken and compared with DA solution prepared in different buffer solutions using SWV technique.
Figure 2. A) Differential pulse voltammograms of 1 mM DA solutions obtained at a potential range of -0.4/+0.5 V on the PGE/CTP/MWCNT electrode surface. B) Square wave voltammograms of 1 mM DA solutions obtained at a potential range of -0.4/+0.5 V on the PGE/CTP/MWCNT electrode surface. The measurements were performed in PBS, pH 7.0. vs. Ag/AgCl/(3 M KCl). Scan rate was 50 mV s-1, pulse size was 50 mV s–1, frequency 25 Hz.
3.2 Effect of solution pH
The effect of solution pH is the essential parameter for the determination of DA. The pH 7.4 which is the physiological pH of the human bodies, so at different pH range of 6.0-8.0 by SWV technique was investigated. The response of PGE/CTP/MWCNT electrode towards DA (1 mM) at different pH was shown in Fig. 3.
Figure 3. Overlaying SWV voltammograms of 1 mM DA solutions obtained at a potential range of -0.4/+0.5 V on the PGE/CTP/MWCNT electrode surface to determine the optimum pH in 0.1 M PBS. (a) pH 6.0, (b) pH 7.0, (c) pH 8.0. The pulse size was 50 mV s–1 and frequency 25 Hz.
3.3. The effect of using different buffer solution
The pH of the solution on the performance of PGE/CTP/MWCNT electrode for DA was investigated at different pH range of 2.0-12.0 by SWV technique. The response of PGE/CTP/MWCNT electrode towards DA (1 mM) at different pH was shown in Fig. 4. DA gave their highest anodic current responses at pH 6.0, and the response was lower at higher pH values.
Figure 4. Overlaying SWV voltammograms of 1 mM DA solutions obtained at a potential range of -5.0/+0.7 V on the PGE/CTP/MWCNT electrode surface to determine the optimum pH in 0.1 M BR buffer. (a) pH 2.0, (b) pH 3.0, (c) pH 4.0, (d) pH 5.0, (e) pH 6.0, (f) pH 7.0, (g) pH 8.0, (h) pH 9.0, (i) pH 10.0, (j) pH 11.0, (k) pH 12.0. Pulse size was 50 mV s–1, frequency 25 Hz.
The effect of changing the buffer type was investigated on DA oxidation in the presence of BR buffer pH 6.0 and PBS pH 7.0. It was clear that PGE/CTP/MWCNT electrode shows relatively better response in BR buffer. DA gave oxidation peak current values of 49.1 μA and 65.6 μA, in the case of BR buffer solution and PBS, respectively (Fig. 3 and 4). When the currents in PBS and BR buffer solution at these pH values were compared, it was decided to continue the study with PBS, pH 7.0.
3.6. Optimization of SWAdSV parameters
Current measurements were carried out using SWAdSV in the potential range from -0.5 to +0.5 V. The following instrumental parameters were used to record the square wave adsorptive stripping voltammograms: Pulse size 50 mV, step size 2 mV and frequency 25 Hz. All the electroanalytical measurements were applied at room temperature. The voltammograms obtained because of the measurements taken between 0 and 180 seconds under optimum conditions were overlayed in Fig. 5A. From the graph (Fig. 5B) drawn between peak current versus accumulation time, 60 second was determined that the appropriate accumulation time for this study.
Figure 5. A) Overlaying SWAdSV voltammograms of 1 mM DA solution prepared in 0.1 M PBS (pH=7.0) buffer, using PGE/CTP/MWCNT, in the potential range of -0.5/+0.5 V, for different accumulation times (0, 15, 30, 45, 60, 75, 90, 105, 120, 150, 180 s). B) Graph plotted with peak current values against accumulation time.
3.6. Quantification of DA using SWAdSV
After the optimum conditions were determined, measurements were taken using DA solutions prepared in 0.1 M PBS (pH 7.0) at different concentrations. The overlayed voltammograms taken between 1mM and 1 µM on the PGE/CTP/MWCNT electrode surfaces are given in Fig. 6A.
Figure 6. A) Overlaying SWAdSV voltammograms of different concentrations of DA using PGE/CTP/MWCNT in 0.1 M PBS (pH 7.0) buffer at a potential range of -0.4/+0.4 V (a) 1.0×10-3 M, (b) 7.5×10-4 M, (c) 5.0×10-4 M, (d) 2.5×10-4 M, (e) 1.0×10-4 M, (f) 7.5×10-5 M, (g) 5.0×10-5 M, (h) 2.5×10-5 M, (i) 1.0×10-5 M, (j) 7.5×10-6 M, (k) 5.0×10-6 M, (l) 2.5×10-6 M, (m) 1.0×10-6 M. The measurements were performed in 0.1 M PBS, pH 7.0, vs. Ag/AgCl/(3 M KCl). Pulse size was 50 mV s–1, frequency 25 Hz, accumulation time 60 s, stirring rate 300 rpm. B) Linear relationship between peak currents and different concentrations of DA.
Calibration graphs were drawn with the data obtained from the measurements taken between 1 mM and 1 µM on the PGE/CTP/MWCNT electrode surface, at concentration ranges of 1x10-6 to 1x10-5 M (Fig. 6B). As seen from the illustration of Fig. 6A, the regression equation could be expressed by the equation: [Ip (μA) =0.2776C + 0.0598 (R2=0.984, n=5)] where Ip: is the adsorptive stripping peak current, C: DA concentration, R: the correlation coefficient, and n: the number of experiments.
4. Conclusions
In the current research, the PGE/CTP/MWCNT electrode prepared by us has been used for the quantitative determination of DA. The developed sensor electrode is sensitive to DA has been observed. As a result, fast, low-cost, and reliable determination of dopamine by SWAdSV technique using PGE/CTP/MWCNT electrode has been demonstrated. In the continuing study, we are going to focus on the quantitative determination of DA in natural samples.
Authors’ contributions
Experiment and drafting of the manuscript, Ş.K.; Operations of directing, controlling, interpreting the results and checking of the manuscript, İ.E.M.; Supervision and final checking of the manuscript, A.D.M.
Acknowledgements
Research Foundation of Necmettin Erbakan University, Konya, Turkey
Funding
We would like to thank to the Research Foundation of Necmettin Erbakan University, Konya, Turkey for financial support of this work.
Availability of data and materials
All data will be made available on request according to the journal policy.
Conflicts of interest
The authors have declared that no competing interests exist.
References
1. Hu, S.; Huang, Q.; Lin, Y.; Wei, C.; Zhang, H.; Zhang, W.; Guo, Z.; Bao, X.; Shi, J.; Hao, A. Reduced graphene oxide-carbon dots composite as an enhanced material for electrochemical determination of dopamine. Electrochim. Acta. 2014, 130, 805–809
2. Wu, Q.; Reith, M.E.A.; Wightman, R.M.; Kawagoe, K.T.; Garris, P.A. Determination of release and uptake parameters from electrically evoked dopamine dynamics measured by real-time voltammetry, J. Neurosci. Methods. 2001,112, 119–133.
3. Zhou, W.H.; Wang, H.H.; Li, W.T.; Guo, X.C.; Kou, D.X.; Zhou, Z.J.; Meng, Y.N.; Tian, Q.W.; Wu, S.X. Gold nanoparticles sensitized ZnO nanorods arrays for dopamine electrochemical sensing. J. Electrochem. Soc. 2018, 165 (12), G3001–G3007.
4. Al-Salahi, N.O.A.; Hashem, E.Y.; Abdel-Kader, D.A. Spectrophotometric methods for determination of dopamine hydrochloride in bulk and in injectable forms. Spectrochim. Acta A Mol. Biomol. Spectrosc. 2022, 278, 121278–121304.
5. Devaraj, M.; Saravanan, R.; Deivasigamani, R.; Gupta, V.K.; Gracia, F.; Jayadevan, S. Fabrication of novel shape Cu and Cu/Cu2O nanoparticles modified electrode for the determination of dopamine and paracetamol. J. Mol. Liq. 2016, 221, 930–941.
6. Reddy, S.; Kumara Swamy, B.E.; Jayadevappa, H. CuO nanoparticle sensor for the electrochemical determination of dopamine. Electrochim. Acta. 2012, 61, 78–86.
7. Park, J.Y.; Myung, S.W.; Kim, I.S.; Choi, D.K.; Kwon, S.J.; Yoon, S.H. Simultaneous measurement of serotonin, dopamine, and their metabolites in mouse brain extracts by high-performance liquid chromatography with mass spectrometry following derivatization with ethyl chloroformate. Biol. Pharm. Bull. 2013, 36, 252–258.
8. Zhang, X.; Zhu, Y.; Li, X.; Guo, X.; Zhang, B.; Jia, X.; Dai, B. A simple, fast, and low-cost turn-on fluorescence method for dopamine detection using in situ reaction, Anal. Chim. Acta. 2016, 944, 51–56.
9. Li, J.; Yang, J.; Yang, Z.; Li, Y.; Yu, S.; Xu, Q.; Hu, X. Graphene–Au nanoparticles nanocomposite film for selective electrochemical determination of dopamine. Anal. Methods. 2012, 4, 1725–1728.
10. Molaakbari, E.; Mostafavi, A.; Beitollahi, H. Simultaneous electrochemical determination of dopamine, melatonin, methionine, and caffeine. Sens. Actuators B Chem. 2015, 208, 195–203.
11. Roushani, M.; Shamsipur, M.; Rajabi, H.R. Highly selective detection of dopamine in the presence of ascorbic acid and uric acid using thioglycolic acid capped CdTe quantum dots modified electrode. J. Electroanal. Chem. 2014, 712, 19–24.
12. Gilbert, O.; Kumara Swamy, B.E.; Chandra, U.; Sherigara, B.S. Simultaneous detection of dopamine and ascorbic acid using polyglycine modified carbon paste electrode: A cyclic voltammetric study. J. Electroanal. Chem. 2009, 636, 80–85.
13. Çelik, H.H.; Özcan, S.; Demir Mülazımoğlu, A.; Yılmaz, E.; Mercimek, B.; Çukurovalı, A.; Yılmaz, İ.; Solak, A.O.; Mülazımoğlu, İ.E. The synthesis of a novel DDPHC diazonium salt: Investigation of its usability in the determination of phenol and chlorophenols using CV, SWV and DPV techniques. Inorg. Chem. Commun. 2020, 116, 107893.
14. Durmuş, N.; Yılmaz, E.; Demir Mülazımoğlu, A.; Mercimek, B.; Çukurovalı, A.; Yılmaz, İ.; Solak, A.O.; Mülazımoğlu, İ.E. A novel highly sensitive carbon based HMPD/GC sensor electrode: copper ions analysis in flour and water samples. Desalin. Water Treat. 2018, 112, 34–41.
15. Demir Mülazımoğlu, A.; Yılmaz, E.; Mülazımoğlu, İ.E. dithiooxamide modified glassy carbon electrode for the studies of non-aqueous media: Electrochemical behaviors of quercetin onto the electrode surface. Sensors, 2012, 12, 3916–3928.
16. Mülazımoğlu, I.E.; Demir Mülazımoğlu, A.; Yılmaz, E. Determination of quantitative phenol in tap water samples as electrochemical using 3,3'-diaminobenzidine modified glassy carbon sensor electrode, Desalination. 2011, 268, 227–232.
17. Mülazımoğlu, I.E.; Yılmaz, E. Quantitative determination of phenol in natural decayed leaves using procaine modified carbon paste electrode surface by cyclic voltammetry. Desalination. 2010, 256, 64–69.
18. İncebay, H. A sensitive quantification of agmatine using a hybrid electrode based on zinc oxide nanoparticles. J. Turk. Chem. Soc. Sect. A: Chem. 2018, 5 (3), 1205–1214.
19. Üstündağ, İ.; Erkal, A. Determination of dopamine in the presence of ascorbic acid on digitonin-doped coal tar pitch carbonaceous electrode. Sens. Mater. 2017, 29 (1) 85–94.

This work is licensed under the
Creative Commons Attribution
4.0
License (CC BY-NC 4.0).
Abstract
In this research, PGE/CTP/MWCNT electrode was prepared by modifying a mixture of coal tar pitch (CTP) and multi-walled carbon nanotube (MWCNT) onto the pencil graphite electrode (PGE) surface. Differential pulse voltammetry (DPV), square wave voltammetry (SWV) and square wave adsorptive stripping voltammetry (SWAdSV) techniques were used independently for standard dopamine (DA) determination. For the determination of DA on the sensor electrode surface, pH study was performed using BR buffer solution and phosphate buffer solution (PBS). For the determination of DA at different concentrations, DPV, SWV and SWAdSV techniques were used in PBS at pH 7.0. Studies were also carried out to determine time and concentration parameters using SWAdSV. Calibration graphs were drawn between 1 mM and 1 µM on the PGE/CTP/MWCNT electrode surface for the DA solutions. As a result of the studies, it has been determined that DA can be analyzed with high sensitivity, fast, and low cost by using the PGE/CTP/MWCNT sensor electrode.
Abstract Keywords
Dopamine, sensor electrode, PGE/CTP/MWCNT, SWAdSV SWV, DPV.

This work is licensed under the
Creative Commons Attribution
4.0
License (CC BY-NC 4.0).
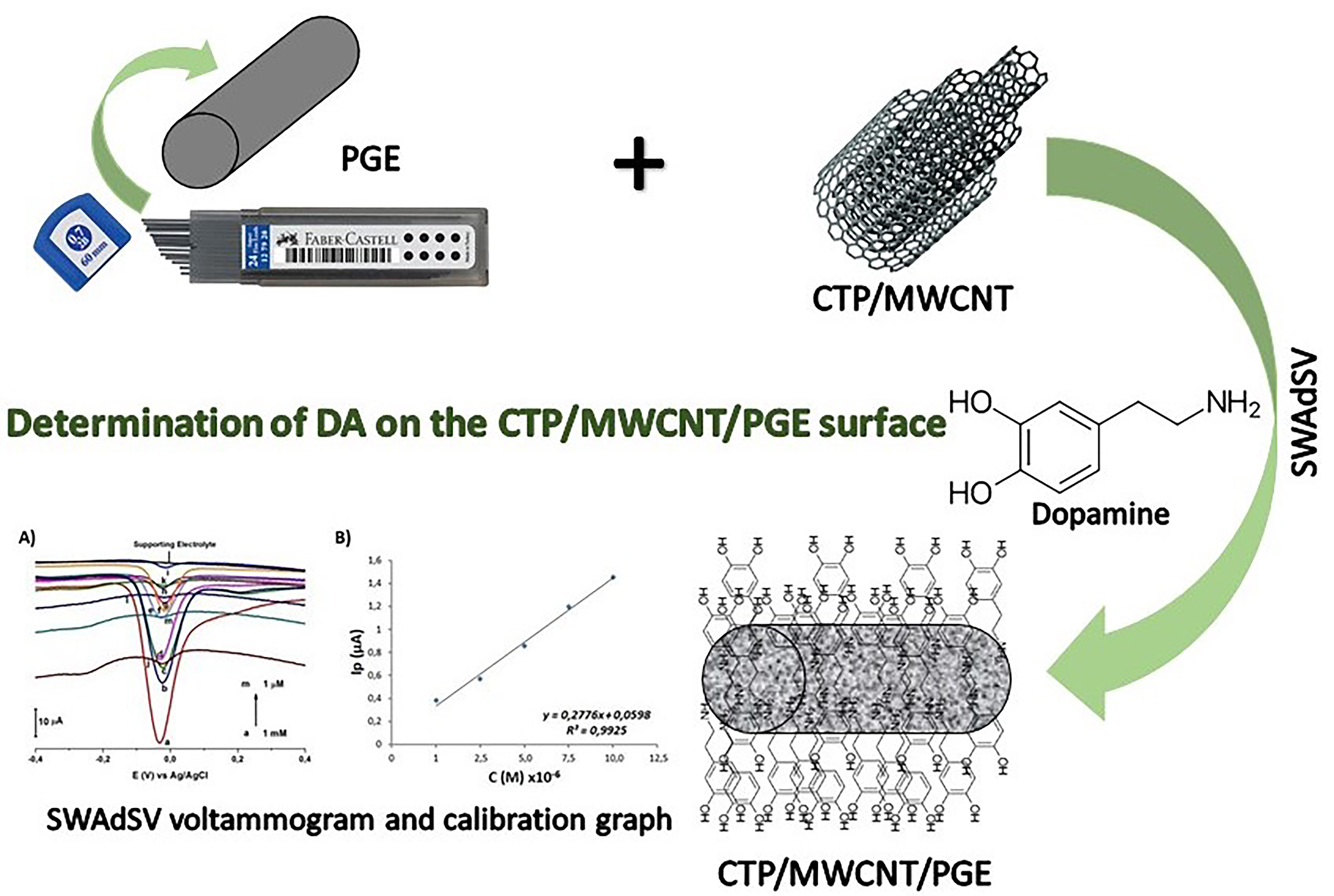
Editor-in-Chief

This work is licensed under the
Creative Commons Attribution 4.0
License.(CC BY-NC 4.0).